Short-Lived Gravitational Instability in Isolated Irradiated Discs
Disc Evolution
Figure 1 compares the evolution of gravitational instabilities in a 0.1 Solar mass disc modelled with live radiative transfer (left panel) and \(\beta \)-cooling (right panel).
\(\beta \)-cooling -- In the absence of irradiation, the disc continuously cools until strong spiral structures develop. Eventually the disc reaches a steady state (in terms of spiral structures) as PdV work and shock heating balance the cooling.
Radiative Transfer -- The inclusion of irradiation essentially fixes the temperatures in the disc since the thermal effects of the star dominates. Hence in an irradiated disc, changes in the surface density instead drive the evolution of the disc. Angular momentum transport by the spiral arms reduces the surface density in the outer gravitationally unstable regions of the disc. Thus, unlike with \(\beta \)-cooling the disc becomes more stable over time.
The Importance of the Star
Figure 2 compares the temperature structure for a 0.1 Solar mass disc modelled with live radiative transfer (left panels) and \(\beta \)-cooling (right panels).
\(\beta \)-cooling -- In the absence of irradiation, the spiral arms are the only source of heat through PdV work and shock heating. Hence, the temperature structure is identical to the density structure.
Radiative Transfer -- The temperature is now controlled by the irradiation of the star as evident by the smooth temperature profile. In contrast to the density structure, the spiral arms are barely visible in the temperature structure when stellar irradiation is included. PdV work and shock heating is negligible compared to the irradiation of the star.
⭐ Hence, an irradiated gravitationally unstable disc cannot regulate gravitational instabilities by adjusting the temperatures in the disc due to the radiation of the star being the dominant (and constant) source of energy.
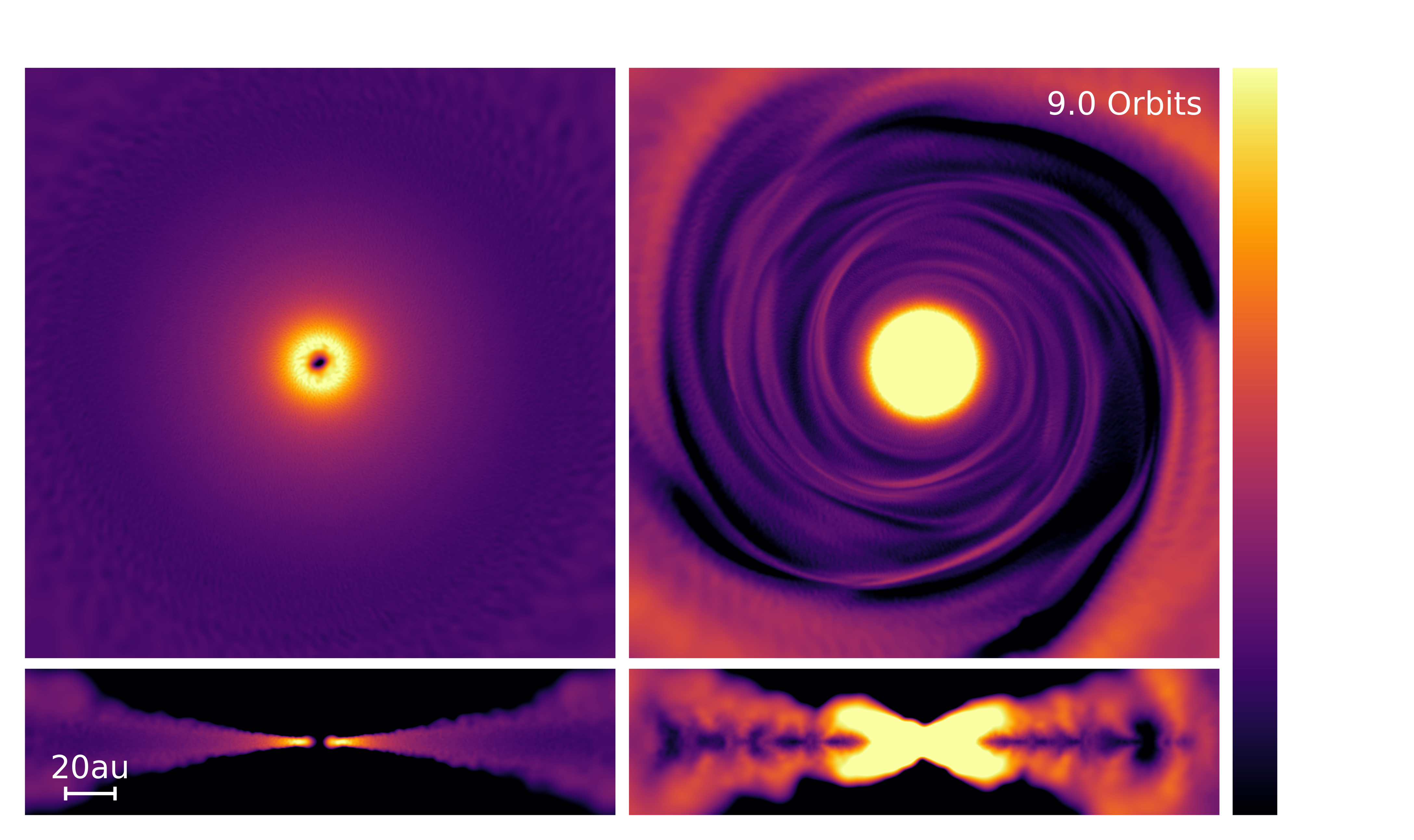
Fragmentation in Higher Mass Discs
Figure 3 shows the final state of a 0.25 Solar mass disc modelled with live radiative transfer. Despite gravitational instabilities being weaker, the disc has fragmented, forming clumps.
In a \(\beta\)-cooled disc, fragmentation could be prevented as the disc could regulate its temperatures through PdV work and shock heating in the spiral arms.
However as shown above, this is negligible in an irradiated disc where the temperatures are set by the star since the thermal effects of stellar irradiation dominates. The dominance of stellar irradiation prevents the disc from stabilising due to PdV work and shock heating in the spiral arms.
⭐ Hence, fragmentation remains a possible fate for irradiated gravitationally unstable discs, confirming the predictions in Kratter & Murray-Clay (2011).
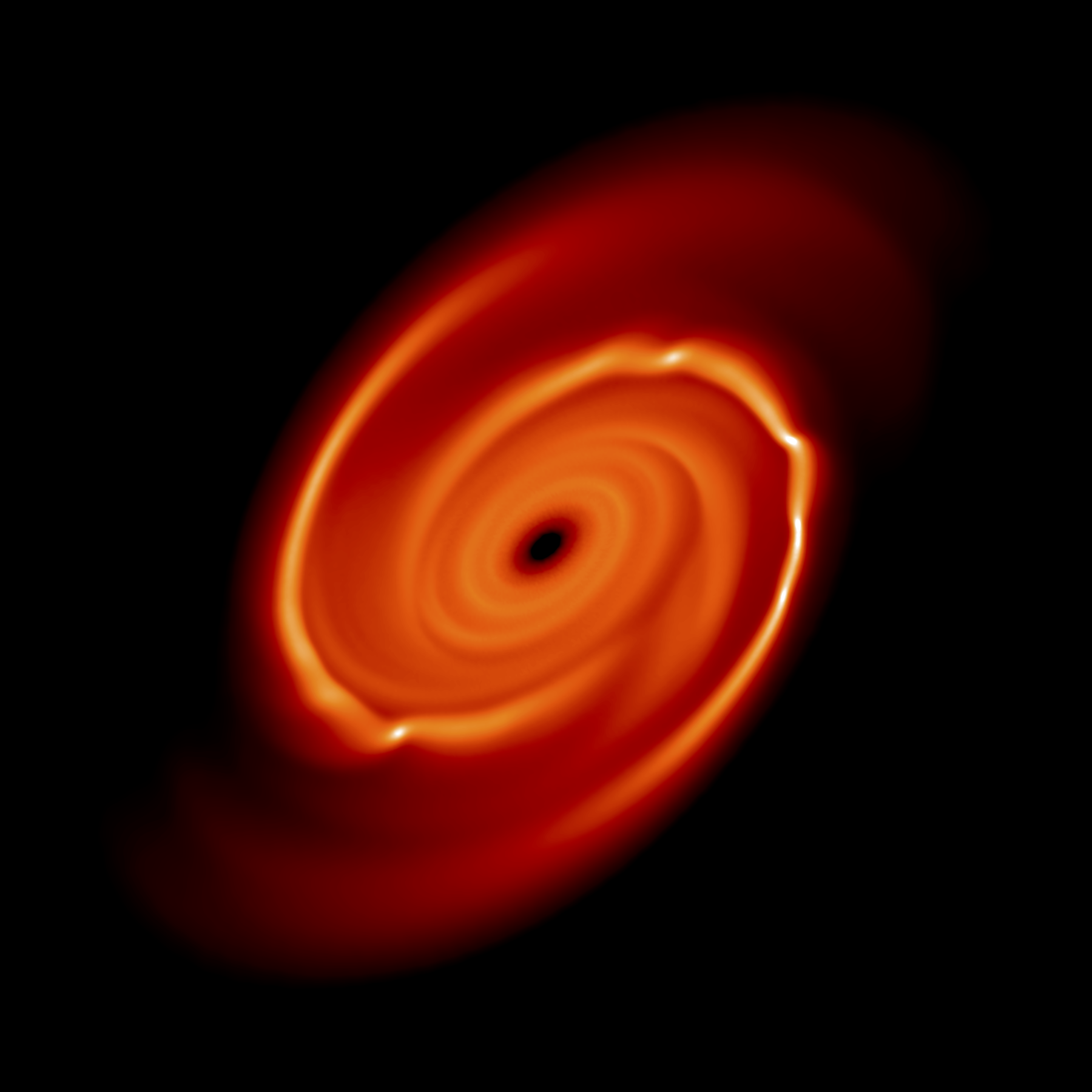